The Science of Drift and Dip
How much do we really know about how spinners move the ball in the air?
Last week, England’s Test tour in Pakistan culminated in a 2-1 series defeat, with the media coverage dominated by discussion of the pitch and spin bowling. Noman Ali and Sajid Khan took 39 of the 40 English wickets to fall in the last two tests, a quite incredible feat for a bowling pair, whatever the conditions.
I’ve been wanting to write about spin bowling for a while, mainly because of some really interesting discussions(/good natured arguments) Sam and I have had this summer around the untested aerodynamic factors at play. This was somewhat sparked by Shoaib Bashir’s selection earlier this year, with talk of the England management group watching clips on social media and being impressed by his height and the amount of ‘action’ he generates on the ball.
Bashir’s tour of India was certainly impressive for someone so young, playing against some of the world’s best players of spin. His two 5-wicket hauls in India are only matched by Fred Titmus, who took his in 1964. The faith shown by Stokes and McCullum was certainly rewarded, but questions remained about his effectiveness in less spin friendly conditions, especially given his poor County Championship record.
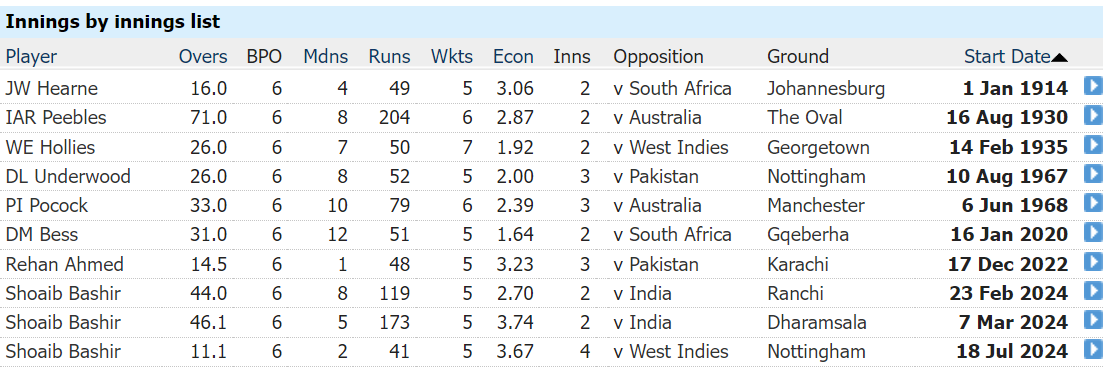
Against the West Indies this summer, he took another 5-fer, and seems to have cemented his place as England’s frontline spinner. While he played second fiddle to Jack Leach in Pakistan, there is a focus on the upcoming away Ashes series, as there always is with England, and Bashir seems to be a key part of the Test plan for Australia.
So today, I thought I’d use Bashir as an example to talk about the pre-bounce movement he gets on the ball, and which factors actually impact the ball’s motion in the air. Himanish Ganjoo also recently wrote a great piece looking at dip in T20 cricket, and its correlation with turn (go and follow him on Twitter and Substack, it is well worth it).
Some of the ideas here follow on from my previous post about Cross-Seam Swing, which has a section on spin at the end (parts of which we are about to refute!). So, buckle up for some never-seen-before, fresh-out-of-the-packet cricket aero theories, slow-motion videos of drift and dip, and the answer to the question: Is everything cool in cricket really just swing bowling? Yes. Yes it is.
Understanding spin axes
I’ve mentioned before that cricket is lightyears behind baseball in term of understanding the mechanics and aerodynamics of the game. While baseball pitchers are using high-speed video to track the speed, orientation and rotation axes of their pitches, in cricket we often simply define deliveries by their direction of turn, e.g. a leg-spinner vs a googly.
Although the late, great Shane Warne was gently mocked for inventing a new variation for every Ashes series he played in, he understood that spinners have more variations at hand than simply which way the ball moves after pitching. Spin rate, rotation axis and seam position all influence how a ball behaves.
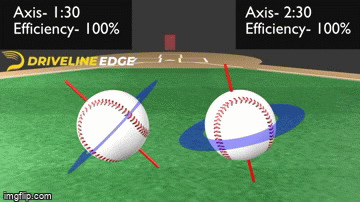
To visualise what I mean by rotation axis, let’s take the baseball example above. On the right, the ball is travelling away from away from us, and spinning clockwise around an almost vertical rotation axis, represented by the red line. The label of Efficiency: 100% refers to the orientation of the red line to the direction of motion, more on that later. On the left, the ball has a similar rotation, but slanted a bit to the left. This is a change in the rotation axis of the pitch, even though the spin rate and pitch direction are the same.
For every cricket delivery, we also have a rotation axis, around which the ball is spinning. If we define a co-ordinate system using the cricket pitch, as is done for ball-tracking technologies, then we can reference the rotation axis to the field of play.
So, let’s take a cricket example to tie this together. Below is a clip from this summer taken from the ECB YouTube channel. Watch closely how the ball is rotating as it travels down the pitch, before turning and hitting off-stump.
This is a classic, right-arm off-spin delivery, with a great seam presentation. But what is the axis of rotation compared to the pitch, and, more importantly, compared to the direction of flight?
In the still above, you can see the ball rotating clockwise around the seam. The rotation axis is pointing away from the camera and is aligned pretty much along the cricket pitch. Watch the video a few times and compare it to the baseball animations above, and hopefully you’ll understand what I mean.
The key takeaway here is that the ball is rotating around an axis pointing in the same direction as the ball is travelling. This is really important for how we expect a ball to move in the air, and is the starting point for our discussion on drift and dip.
The Magnus Effect
I introduced the Magnus Effect in my last piece when discussing curveballs in baseball, or curved free-kicks in football, (or how I hook and slice the ball in golf - SG) but it’s worth mentioning how it works again.
When a ball is spinning, a pressure distribution is generated around the ball which moves it sideways. A classic example is shown in the video below, where a spinning basketball is dropped off a dam. It’s worth taking 3 minutes to watch this, just for some background.
A key feature of the Magnus Effect is that the axis of rotation needs to be at right angles to the direction of travel. If you look at the thumbnail above, the ball is spinning backwards, so the rotation axis is sideways, and the ball is falling downwards. Therefore, the rotation axis is perpendicular to the motion of the ball, creating a force in the direction away from the dam.
This effect is the origin of Spin Efficiency in baseball. It describes how perpendicular the rotation axis and direction of travel are. A perfect right angle gives a 100% spin efficiency, as that creates the most Magnus Force. Essentially, 100% of the spin is creating sideways motion, often called ‘break’ in baseball.
If you look at the cartoon examples of baseballs at the top of the previous section, both have an axis creating 100% efficiency, just slightly tilted to each other. This tilt changes the direction of the force, from being a pure curveball on the right, to a ball with some curve and some lift on the left. (Anyone familiar with the ‘Right Hand Rule’ from school can use this to work out the direction of a Magnus Force with 100% efficiency)
Parallel rotation axes don’t create a Magnus Force
As you may well have spotted, the Bashir delivery above doesn’t fit the Magnus Effect criteria. In fact, it has almost 0% spin efficiency, so minimal drift is created - you can see this in the video. There isn’t even any dip created, as that requires top-spin, which would require the ball to be rotating clockwise away from the camera.
What we have, instead, is rifle-spin, also called gyro in baseball, which creates no Magnus Force*. I know this is contrary to what I said in my last blog, but hey, learning is fun. It’s important to note that not all deliveries are purely rifle-spin. Take a look at another Bashir delivery below.
*Side note for the real nerds (Thanks! - SG): There is actually a small Magnus component generated by the vertical motion of the ball, which drops at an angle of ~20 degrees. This is like the basketball and dam. However, the low vertical velocity and small-ish drop angles mean this is unlikely to create the large horizontal motion we associate with ‘drift’.
The rotation axis on this delivery is a bit harder to identify as the seam is wobbling slightly, but it is much more top-spin than rifle-spin. This, and the subsequent Magnus Force, help create the ‘drop’ that Atherton mentions on commentary.
This change in axis is quite common among off-spin bowlers, Nathan Lyon is often noted for the dip he creates in his stock delivery. However, there is still almost no sideways component of the Magnus Force in the above example, so we need another way to explain the drift motions we see.
Sideways drift is caused by the seam, rather than rotation
So how do spinners actually generate drift if not via the Magnus Effect? The answer, unsurprisingly on the Science of Swing blog, is in the boundary layers on the ball. Let’s looks at some examples below.
The easiest case of sideways movement is a good, old-fashioned arm-ball, which is basically a slow swinging delivery, set up the same way a seamer would. The seam is angled toward the slip cordon, with the shiny side of the ball on the non-seam side, and the ball swinging in the direction which the seam is pointing. Joe Root has a great arm-ball and if you watch this video on the ECB Twitter page, you can see how much the ball swings before it bounces.
However, what about a standard off-break delivery, which drifts away, before turning in? Below is a still of a Nathan Lyon stock ball, where you can see that the rotation axis is mid-way between the two examples from Bashir. This delivery is majority rifle spin, but tilted slightly so that the seam is slightly ahead of the midpoint on the right side of the image. This is the key to drift.
What happens here is very similar to swing, where the seam alters the boundary layer separation on each side, except here, the seam affects both sides of the ball. I mentioned previously Barton Smith’s great work on ‘Seam Shifted Wake’ pitches, where the separations ‘fix’ to the seams, and the same thing happens for spinners.
On the left hand side of the ball in the image above, the seam is slightly behind centre, whereas on the right, it is slightly in front. If the boundary layer separates on the seam in both cases, there will be a separation asymmetry, shifting the air behind the ball to the right, and moving the ball to the left. This is a similar mechanism to the Cross Seam Swing delivery in my previous post.
The same thing happens for a leg-spin delivery drifting in the opposite direction. Have a watch of this beauty from Adil Rashid, and see how the seam is angled slightly in front of the midpoint on the right-hand side this time (this one like Warne’s ball of the century, except in HD - SG). The clip is from an ECB video on big spin with some more great examples to watch if you have a spare 10 minutes.
The complexity of spin variations is still to be explored
It is worth saying that a lot of these ideas are currently untested, and need refinement. I’ve already discussed how this idea works for spinners bowling ‘over the seam’, where the seam isn’t in the plane of rotation, in my last post, but I hadn’t realised that the mechanisms work for almost any spin delivery.
Sam, myself and Sam’s new Masters Student Zeynep, are looking to test some of these ideas more rigorously in a wind tunnel, and hope soon to have infrared images to illustrate the cases I’ve discussed. Hopefully this will shed light on the optimal ball orientation for achieving different types of movement in the air.
The same mechanism of a slightly off-centre seam can also be applied to create dip by tilting the seam forwards. Simply view the diagram above as a side-on view, rather than a top-down one, and you can see how it would work.
I could probably run through 50 more examples, but I’ll leave things here for now and save some content for later. I hope this has been interesting and you join me and Sam in paying closer attention to the seam position when spinners are bowling!
Go well!
so if magnus doesn't explain drift of a spinner, how did Kuhnemman got reverse drift when operating at higher speeds?
The one thing I didn't get is that the drift maximisation should occur when the boundary layer separation distance is maximum between both sides of the ball from each other, so it should be somewhere close to the top spinner, but in practice it is closer to the square spinner. So maybe the optimal width of wake formation and resultant reaction force is dependent on maximising the horizontal distance between both points which occurs closer to the square seam/rifle spin seam?
Another thing that can be inferred is, does this necessitates the seam to be upright and there wouldn't be much drift on the scramble seam even if the rotational axis is kept the same as Lyon's. Unlike for dip?